Understanding Quantum Entanglement: A Deep Dive into the Fabric of Reality
Quantum entanglement is one of the most intriguing and perplexing phenomena in the realm of quantum physics. It challenges our understanding of the nature of reality, information, and the interconnectedness of particles in the universe. In this blog post, we will explore the fundamental principles of quantum entanglement, its implications, the experiments that have tested its validity, and its potential applications in technology and philosophy.
What Is Quantum Entanglement?
At its core, quantum entanglement describes a special physical phenomenon that occurs when two or more particles become interconnected in such a way that the state of one particle cannot be described independently of the state of the other(s), even when the particles are separated by vast distances. This interdependence exists regardless of the space between them, leading to what Einstein famously referred to as “spooky action at a distance.”
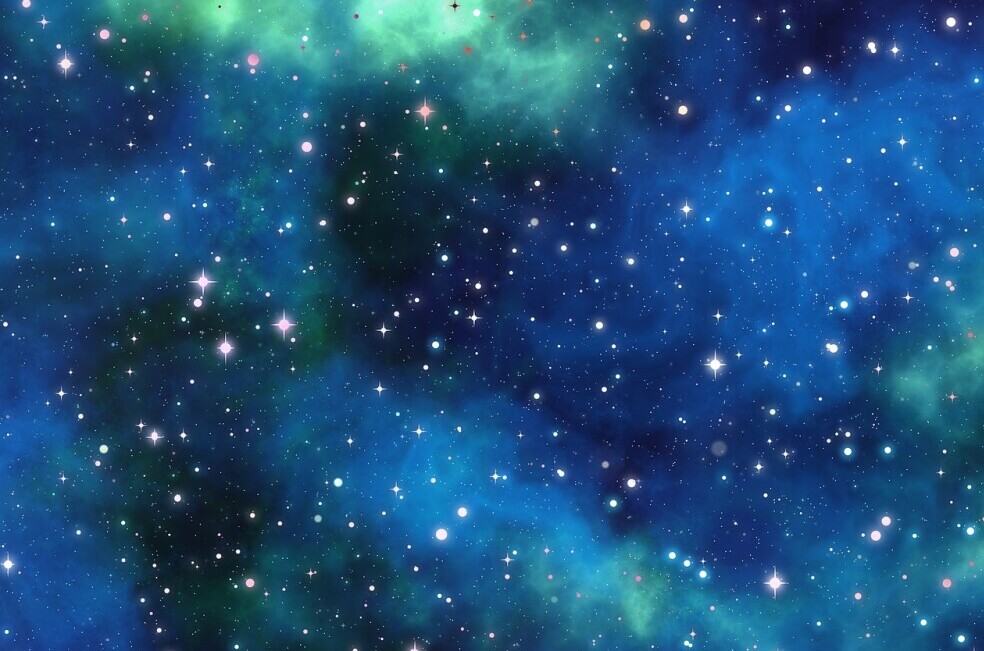
The Foundations of Quantum Mechanics
To appreciate quantum entanglement, it is essential to revisit the principles of quantum mechanics. Quantum mechanics is the branch of physics that deals with the behavior of matter and light at the atomic and subatomic levels. Some foundational elements of quantum mechanics include:
- Wave-Particle Duality: Particles such as electrons exhibit both wave-like and particle-like behaviors, depending on how they are observed.
- Superposition: Quantum particles can exist in multiple states simultaneously until they are measured. For instance, an electron can spin in multiple directions at once until we observe it.
- Uncertainty Principle: Formulated by Werner Heisenberg, this principle states that certain pairs of physical properties (like position and momentum) cannot be simultaneously measured with arbitrary precision.
The Birth of Entanglement
The concept of quantum entanglement was introduced by Albert Einstein, Boris Podolsky, and Nathan Rosen in a 1935 paper famously known as the EPR paradox. The EPR paper sought to illustrate what they considered to be the inadequacies of quantum mechanics—specifically, its incompleteness—by proposing that if quantum mechanics is complete, then entangled particles could affect one another instantaneously, raising questions about locality and reality.
Understanding Entanglement Through an Example
Consider a pair of entangled particles, such as two photons. When these particles are produced, they enter an entangled state, which means that their properties (like polarization and spin) are correlated. If you measure the polarization of one photon and find it to be vertical, the other photon, regardless of the distance separating them—whether a few meters or light-years—will instantly be confirmed to have horizontal polarization. This correlations defy classical expectations and showcase a fundamental aspect of quantum mechanics.
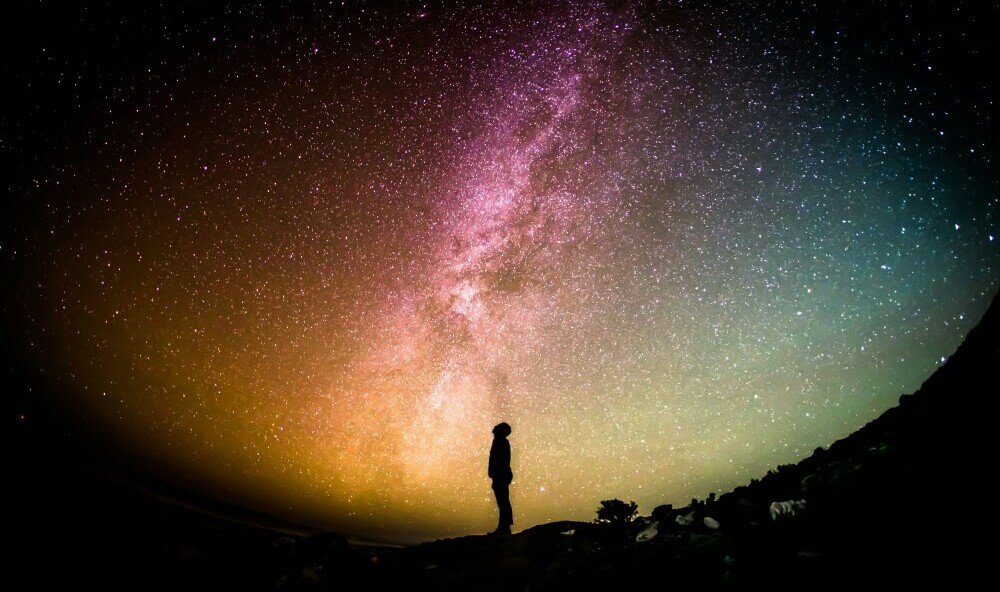
Bell’s Theorem and Experimental Validation
To delve deeper into the implications of quantum entanglement and to address the EPR paradox, physicist John Bell formulated what is now known as Bell’s Theorem in the 1960s. Bell’s Theorem presents inequalities (Bell inequalities) that test whether the outcomes of measurements on entangled particles can be explained by local hidden variables—essentially, predetermined conditions.
Numerous experiments have since been conducted to test Bell’s theorem. The most famous of these experiments, starting in the 1970s, consistently demonstrated violations of Bell’s inequalities, thus supporting the non-local nature of quantum mechanics and the validity of quantum entanglement. These results have shifted our understanding of the universe, suggesting that particles do not adhere to the classical notion of separate, individual realities.
The Implications of Quantum Entanglement
Quantum entanglement has profound implications across various domains:
- Quantum Computing: At the heart of quantum computing lies entanglement. Quantum computers exploit entangled states, allowing them to process information in ways that classical computers cannot, potentially solving complex problems exponentially faster.
- Quantum Cryptography: Quantum key distribution (QKD) uses the principles of entanglement to create secure communication channels. Eavesdropping on an entangled system alters the state of the particles, thus revealing the presence of an intruder.
- Quantum Teleportation: Though often sensationalized in science fiction, quantum teleportation is a real and well-studied process relying on entanglement to transfer quantum states between particles without transmitting the physical particles themselves.
- Philosophical Implications: Quantum entanglement raises fundamental questions about the nature of reality and the interconnectedness of all things. It challenges classical notions of separateness and locality, provoking debates in the philosophy of science.
Challenges and Misinterpretations
Despite its fascinating implications, quantum entanglement is often misunderstood. Some common misinterpretations include the idea that it allows for faster-than-light communication or the assumption that it entails a form of nonlocality without any constraints. However, the no-signaling theorem proves that while entangled particles exhibit correlated behaviors, it is impossible to use this phenomenon to transmit information instantaneously.
Conclusion
Quantum entanglement stands as one of the most remarkable features of quantum mechanics, challenging our understanding of the universe at a fundamental level. Through entanglement, we glimpse a reality that is intricately connected, where observation influences the nature of existence. From advancing technologies like quantum computing and cryptography to inspiring philosophical inquiries about the nature of reality, quantum entanglement continues to be a pivotal topic in both science and philosophy. As research progresses, we may uncover deeper insights into the fabric of reality, shedding light on the profound mysteries that define our universe.
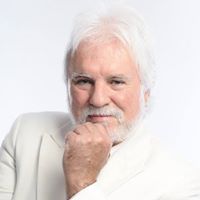
Grant Edward Rayner