Understanding Quantum Physics: A Journey into the Microscopic Realm
Quantum physics, also known as quantum mechanics, is one of the cornerstones of modern physics, fundamentally altering our understanding of nature at its most basic level. Emerging in the early 20th century, this revolutionary field of study challenges our classical perceptions of the universe, introducing concepts and principles that may seem counterintuitive. In this blog post, we will explore the fundamental principles of quantum physics, its historical development, key experiments, and its implications for technology and the future.
A Brief History of Quantum Physics
The origins of quantum physics can be traced back to the late 19th and early 20th centuries, a period marked by significant advancements in our understanding of electricity, magnetism, and thermodynamics. Classical physics, based on Newtonian mechanics and Maxwell’s equations, faced challenges in explaining phenomena at the atomic and subatomic levels.
Max Planck and the Birth of Quantum Theory
The seeds of quantum theory were sown in 1900 when German physicist Max Planck proposed that energy is emitted or absorbed in discrete units, or “quanta.” This discrete approach resolved the ultraviolet catastrophe problem associated with black-body radiation. Planck’s constant (h) became a fundamental element in quantum mechanics, setting the stage for a transformational journey into the microcosm.
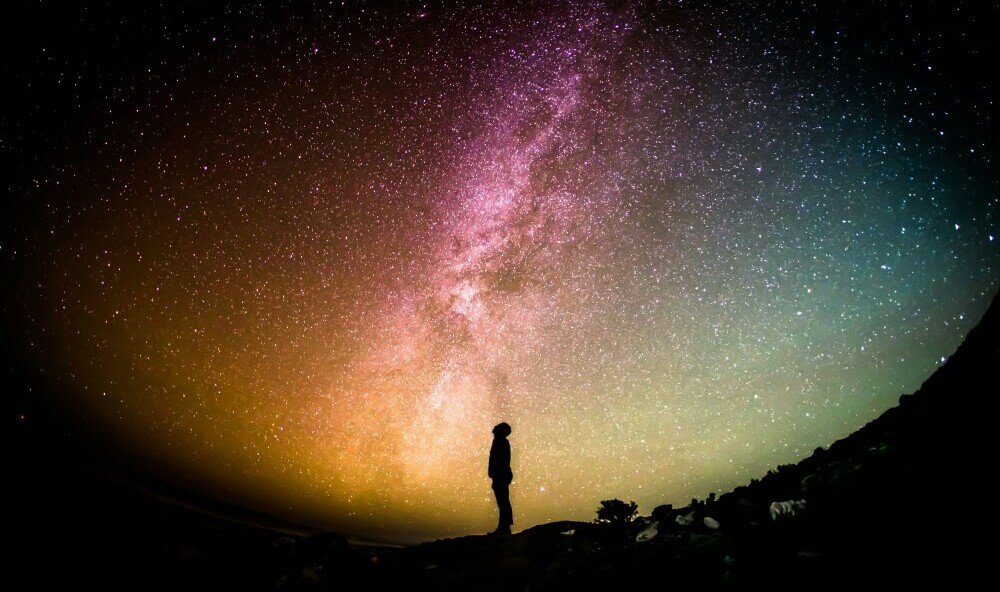
Albert Einstein and the Photoelectric Effect
In 1905, Albert Einstein expanded upon Planck’s ideas with his explanation of the photoelectric effect—demonstrating that light can behave as both a wave and a particle. Einstein postulated that light consists of packets of energy, or photons, which can dislodge electrons when striking a metal surface. This duality of light played a pivotal role in establishing quantum theory as a robust scientific framework.
Niels Bohr and the Hydrogen Atom
In 1913, Niels Bohr introduced his model of the hydrogen atom, positing that electrons occupy quantized energy levels. This model successfully explained spectral lines of hydrogen and marked a significant step towards integrating quantum theory with atomic structure. Bohr’s work was crucial in shaping the understanding of how atoms interact with light and energy.
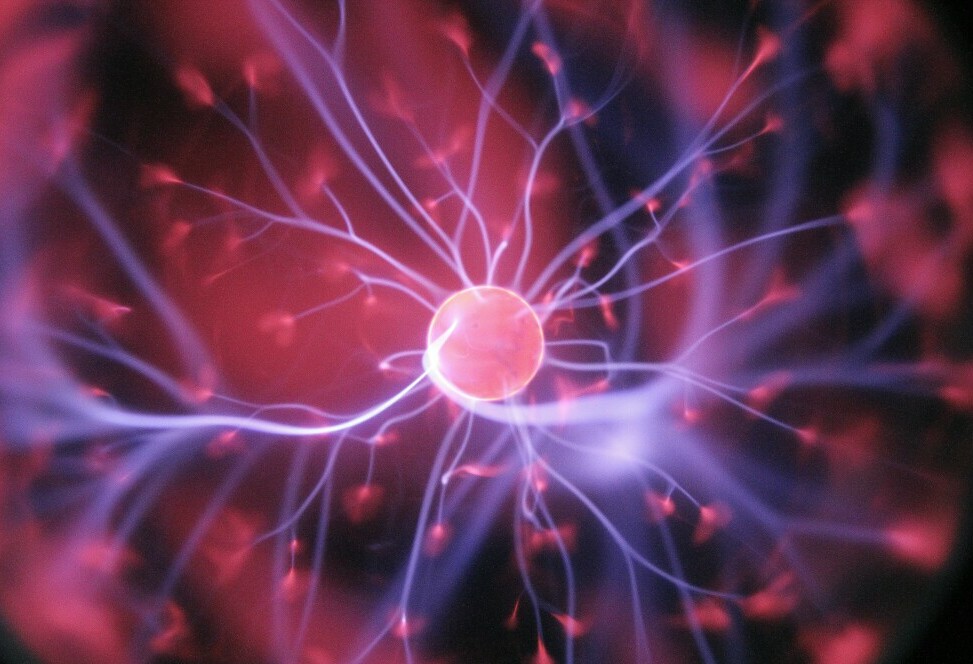
Quantum Mechanics Takes Shape
The 1920s witnessed the simultaneous development of quantum mechanics by several physicists, including Werner Heisenberg, Erwin Schrödinger, and Paul Dirac. Heisenberg’s uncertainty principle articulated the principle that certain pairs of physical properties, like position and momentum, cannot simultaneously be measured with arbitrary precision. Schrödinger introduced the wave equation, describing the behavior of quantum systems as wave functions. The culmination of these efforts led to a complete mathematical framework for quantum mechanics.
Fundamental Principles of Quantum Physics
At the heart of quantum physics are several core principles that distinguish it from classical physics. Here are some of the most important concepts that illustrate the behavior of matter and energy on a microscopic scale.
1. Wave-Particle Duality
One of the most perplexing aspects of quantum physics is wave-particle duality, which posits that particles, such as electrons and photons, exhibit both wave-like and particle-like properties depending on the experimental context. This duality is beautifully exemplified in the double-slit experiment, where light and matter demonstrate interference patterns characteristic of waves when not observed, yet behave as particles when a measurement is made.
2. Quantum Superposition
In quantum mechanics, particles can exist in multiple states simultaneously until a measurement is made. This principle is famously illustrated by Schrödinger’s cat thought experiment, where a cat is both alive and dead until someone opens the box and observes its state. Superposition underscores the probabilistic nature of quantum mechanics—where outcomes exist only as a probability until observed.
3. Entanglement
Quantum entanglement describes a phenomenon where particles become intertwined in such a way that the state of one particle is dependent on the state of another, regardless of the distance separating them. This unique property was described by Einstein as “spooky action at a distance.” Entangled particles can instantaneously affect one another’s states, leading to potential applications in quantum computing and secure communication.
4. The Observer Effect
The observer effect refers to the alteration of a system’s state due to observation or measurement. In quantum mechanics, measuring a property, such as position, can change the state of a particle, emphasizing the role of the observer in determining outcomes. This principle highlights the complex interplay between measurement and reality at the quantum level.
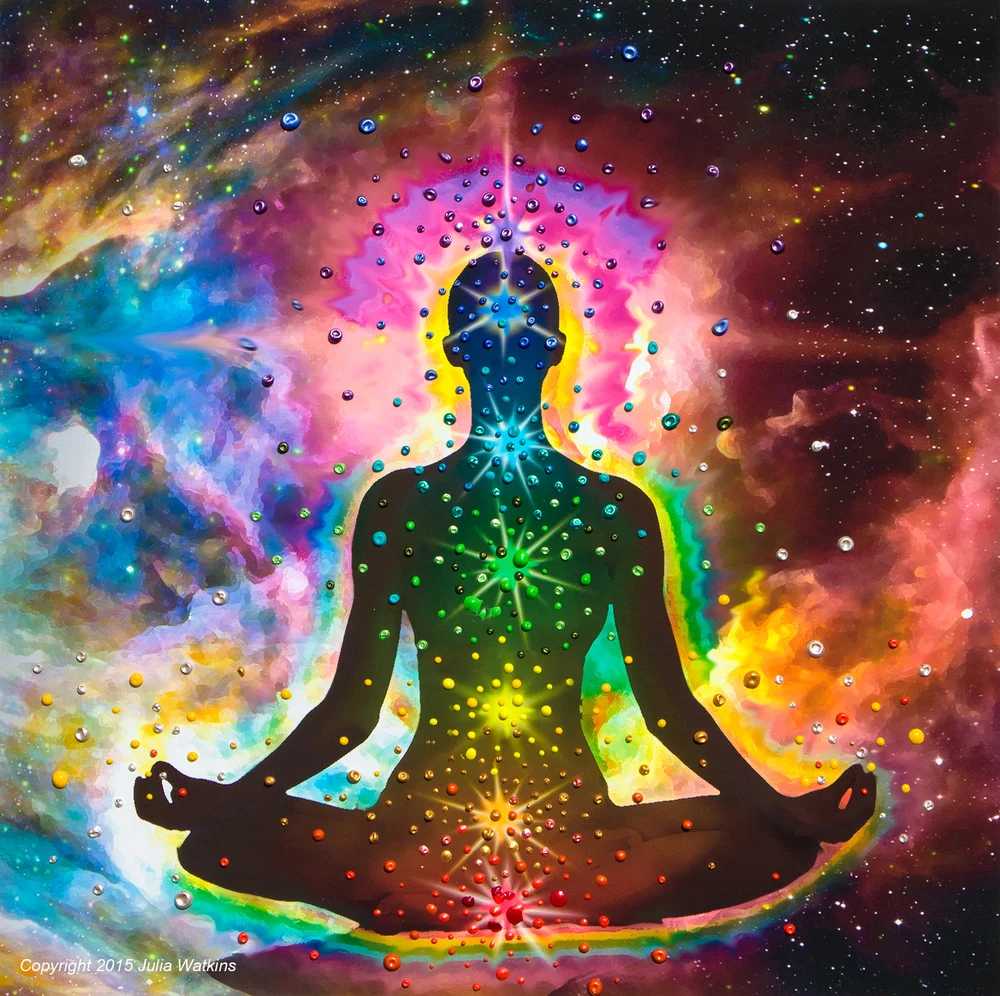
Key Experiments in Quantum Physics
Numerous experiments have validated quantum principles, further entrenching the field within scientific discourse. Here are some pivotal experiments that have shaped our understanding:
The Double-Slit Experiment
First conducted in the early 19th century and revisited in the 20th, the double-slit experiment demonstrates wave-particle duality. When particles are fired at a barrier with two slits, they create an interference pattern on a detecting screen, implying wave behavior. However, if a detector is placed to observe which slit a particle goes through, the interference pattern vanishes, revealing particle-like behavior. This experiment encapsulates the peculiar nature of quantum mechanics.
Bell’s Theorem
In the 1960s, physicist John Bell formulated Bell’s theorem, which highlighted the discrepancies between quantum mechanics and classical physics. Subsequent experiments conducted in the 1970s and beyond have confirmed the predictions of quantum mechanics, demonstrating that entangled particles truly behave in ways that defy classical intuition and support the non-locality inherent in quantum theory.
The Aspect Experiment
Conducted by Alain Aspect and his team in the early 1980s, this series of experiments tested Bell’s inequalities and provided strong support for quantum entanglement. The results demonstrated that entangled particles remained correlated, regardless of the distance separating them, reinforcing the idea that quantum mechanics challenge classical notions of locality.
Applications of Quantum Physics
Quantum physics transcends theoretical interest; it has led to groundbreaking technologies that revolutionize modern life. Here are some notable applications of quantum mechanics:
1. Quantum Computing
Quantum computers leverage the principles of superposition and entanglement to perform calculations at unprecedented speeds. Unlike classical bits, which exist as 0s or 1s, quantum bits (qubits) can exist in multiple states simultaneously, allowing quantum computers to solve complex problems, such as optimization and cryptography, more efficiently than traditional computers.
2. Quantum Cryptography
Quantum cryptography employs quantum mechanics to create secure communication channels. Quantum key distribution (QKD) ensures that any attempt at eavesdropping can be detected, as the act of measuring the quantum states would alter them, alerting the parties involved. This promises a new era of secure communications that classical cryptography cannot offer.
3. Quantum Sensors
Advanced sensors based on quantum principles achieve a level of precision unattainable through classical methods. Quantum sensors can be applied in various fields, from medical imaging to navigation, providing unprecedented accuracy for measurements.
4. Quantum Simulation
Quantum simulators designed to mimic the behavior of quantum systems have significant implications for materials science, chemistry, and condensed matter physics. By simulating complex quantum interactions, researchers can gain insights into new materials and chemical reactions.
The Future of Quantum Physics
Quantum physics continues to be a vibrant area of research, with scientists exploring new theories, improving experimental techniques, and developing novel applications. From the question of whether there are hidden variables that can explain the quirkiness of quantum behavior to the ongoing pursuit of a unified theory that reconciles quantum mechanics with general relativity, the future of quantum physics is vast and filled with possibilities.
Conclusion
Understanding quantum physics not only deepens our comprehension of the universe’s underlying principles but also propels technological advancements that shape our daily lives. The fundamental ideas of wave-particle duality, superposition, entanglement, and the observer effect provide a captivating glimpse into a realm that defies conventional logic.
As ongoing research in quantum mechanics continues to yield new insights and applications, we stand on the threshold of a technological and philosophical revolution—one that challenges our very notions of reality itself. Whether you are a physicist, a student, or simply a curious individual, the world of quantum physics invites you to explore the intricacies of existence at the smallest scales, igniting a sense of wonder about the universe we inhabit.
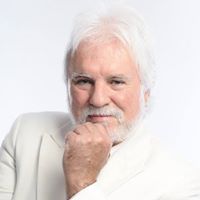
Grant Edward Rayner